Lithium Ion battery and alumina
Applications for High Purity Alumina in Lithium-ion Batteries
Neal White
Introduction
Lithium-ion batteries (LIB) are the
ubiquitous global choice for rechargeable
battery applications, including mobile
phones, Electric Vehicles (EV) and Energy
Storage Systems (ESS). They have been in
production since the 1990’s and
improvements in safety and performance
are continuously being made. Energy
density has increased at around 3% per
year and has now reached approximately
300Wh/kg and 750Wh/l. Despite this
improvement more capacity and longevity
is required to meet the ever-increasing
power demands of the mobile
communication devices and EV
applications. In particular, it is increasingly
important to maximise the cycle life and
minimise the capacity fade of the cells –
this will increase the cumulative capacity
and lifetime of the cells. This in turn
reduces the requirement for new raw
materials to supply the increasing demand
for batteries.
Despite their global usage, current LIB
systems are not fully stable due to
phenomena such as reactions of the
solvents with the anode, cathode structural
changes with cycling, loss of elements from
the cathode compounds, decomposition of
the electrolyte salts and others.
High Purity Alumina (HPA) can play a role in
several crucial aspects of the LIB system to
enhance safety, decrease anode and
cathode degradation reactions and
increase cell lifetime. HPA can be applied to
the battery separator and as coatings on
the graphite (and silicon graphite
composites) anode and on the cathode.
This report will highlight a brief description
of the application of HPA to these three
areas of the cell and the afforded benefit.
AEM addresses this application through the
operation of a clean process run on 100%
renewable energy, certified (pending)
according to ISO9001, 14001 and 45001,
with minimal processing additions –
hydrochloric acid is the only process
chemical, which is recycled from the latter
steps of the process.
A purified secondary product – aluminium
chloride hexahydrate (ACH) is also
produced. The process ensures no foreign
metallic inclusions or transition metal
compounds are present in the product.
HPA as a battery separator coating
A typical lithium-ion battery contains a separator.
LIB separators are essential for the safe and
effective functioning of the cell. They have specific
properties and must be engineered to meet strict
criteria of strength, pore size, thermal resistance,
lithium-ion conductivity etc.
LIB systems are required to be stable and retain
capacity over many cycles of oxidation/reduction
and years of operation, for this reason they
require the use of high purity materials to ensure
adequate performance and safety. Safety issues do
not always manifest during production testing and
may only become apparent themselves after many
cycles of operation. LIBs are susceptible to the
formation of dendrites which can lead to several
problems within the cell, such as a simple loss of
capacity and cycle life for soft short circuits and
severe local heating due to hard shorts, which in
turn can lead to thermal runaway and fire.
The two primary conditions for the formation of
dendrites in lithium-ion batteries is provided as
follows:
a. Deposition of metallic lithium on the
graphite surface on overcharge,
deposition on the graphite of other,
Transition Metals, from the electrolyte
solution under normal charge/discharge
conditions.
b. Transition metals leaching from conventional alumina can be a further source of dendrite initiation, which can be mitigated by high purity alumina, creating a strong value proposition for 4N and 5N products.
In addition to the issue of metal deposition and the formation of dendrites the impact of sodium impurity on the function of LiB is problematic. Traces of sodium can affect the cell capacity and cycle life by its influence on the Solid Electrolyte Interface – SEI- by causing it to thicken and increase in resistance. The AEM process yields very low sodium impurities due to the omission of the sodium hydroxide commonly used in the Bayer process for alumina production.
The application of alumina to the separator as a thin coating confers several performance and safety benefits to the cell.
b. Transition metals leaching from conventional alumina can be a further source of dendrite initiation, which can be mitigated by high purity alumina, creating a strong value proposition for 4N and 5N products.
In addition to the issue of metal deposition and the formation of dendrites the impact of sodium impurity on the function of LiB is problematic. Traces of sodium can affect the cell capacity and cycle life by its influence on the Solid Electrolyte Interface – SEI- by causing it to thicken and increase in resistance. The AEM process yields very low sodium impurities due to the omission of the sodium hydroxide commonly used in the Bayer process for alumina production.
The application of alumina to the separator as a thin coating confers several performance and safety benefits to the cell.
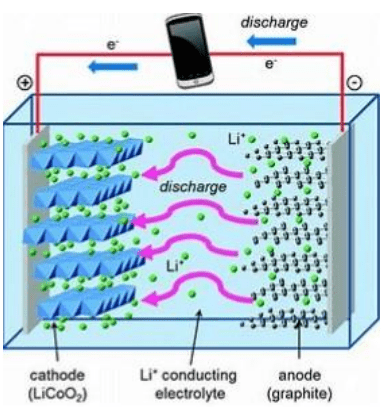
HPA coating for Graphite and Silicon/graphite composite anodes
High purity (4N) graphite is the long-established
anode of choice for LIB. Graphite is widely used
because of its layered structure, high lithium
insertion capacity (372 mAh/g,) high reversibility,
and low cost. In addition, the low potential for Li
insertion produces a high cell voltage, but the fastcharging capability is limited due to the potential
for lithium plating on overcharge.
Anode capacity degradation is partially because of
the reactions between the organic solvents of the
electrolyte and the graphite. The solvent reacts with the anode, forming solid or liquid layer at the
graphite surface, the Solid Electrolyte Interface
(SEI). This results in an irreversible capacity loss
seen on the first cycle. This SEI layer is permeable
and allows transport of ions from the electrolyte
to the graphite surface. The thickness of the SEI
will increase with time which increases the
impedance of the interface and the overpotential
of ions, so it will grow over time, which further
deteriorates the battery and its life span. Failure of
the graphite anode is due to the reduced reaction
rate leading to power fade in batteries and
capacity loss.
One of the methods to mitigate the degradation of
the anode capacity loss is the coating on the anode
particles with alumina. This has the effect of
reducing the contact of the graphite surface with
the electrolyte. This in turn cuts down the reaction
of the electrolyte components with the graphite
and prevents the loss of electrolyte, the build-up
of a thick and increasing SEI. The alumina layer
should be thin, in the order of a few nanometres,
to ensure lithium-ion transport is not impeded.
The alumina should be high purity so as not to add
any problematic elements to the system.
Improvements in cell performance such as a
reduction in irreversible capacity loss and
increased cycle life have been reported.
Battery manufacturers have very strict purity
requirements for graphite to be used in LIB
anodes. A typical purity level is 4N+. To ensure the
overall purity level of the cell system is
maintained, AEM is developing an easy high purity
alumina coating method for graphite using a 4N+
pre-cursor which can be applied at scale and low
cost.
Silicon and silicon/graphite composite anodes also
require protection from degradation via
electrolyte reactions and excessive expansion of
the silicon, which leads to increased surface area,
reaction with the electrolyte and isolation of the
particles from the bulk material and current
collector. In a similar way to the graphite only
anode an alumina coating can protect the silicon
from the electrolyte and increase the lifetime of
the anode. The silicon and silicon/graphite
composite can be treated in a similar process
currently under development by AEM.
HPA coating for LIB Cathodes
Lithium-ion battery cathodes are dominated by
layered lithium metal oxides and
predominately lithium nickel manganese cobalt
oxides (NMC) with varying proportions of Ni
Mn and Co. The industry is moving to high
nickel materials and is reducing the cobalt
content. The high nickel NMC compounds have
good initial capacity, but nevertheless suffer
from degradation during cycling. The loss of
capacity is predominately a result of surface
chemical changes which results in delithiation
and loss of oxygen and a destabilised crystal
structure. A volume change can also take place
which induces cracks in the cathode particles
which exposes more cathode surface to the
electrolyte. In a similar manner to the graphite
the cathode aids the decomposition of the
electrolyte and may lead to surface films and
the production of HF from the
hexafluorophosphate salt. The resulting HF can
dissolve cathode material further degrading
the performance of the cell.
Surface coating of the cathode particles is a
mitigation method to reduce the cathode
surface interacting with the electrolyte.
Coating the cathode powder also helps to
prevent the structural changes in the NMC
material and reduces the microcracking of the
particles. All these mitigations contribute to
increased capacity and cycle-life of the NMC.
Several oxide materials have been investigated
for cathode coating and alumina has been used
successfully by several methods: vapour
deposition, solution and dry mechanofusion
application methods to reduce degradation and improve performance. The coating
thickness is dependent on the porosity of the
coating. Continuous dense locating layers
should be restricted to a few nm to be an
effective barrier without degrading the cell
performance. Porous, more particulate coating
can be several tens of nm thick and give good
results.
As a working hypothesis the mechanism of
protection is primarily surface blocking by the
alumina. Hence, a good, but not complete,
coverage of the cathode particles is required to
affect the long-term improvement in
performance. Ideally, the coating should be an
electronic insulator, and slow down cathode
dissolution without impeding lithium ion
transport to any significant extent.
Electrochemical evaluation of optimized
coatings shows a significant enhancement in
rate performance and capacity retention by
the optimal amount of coating material, while
the solid electrolyte interface (SEI) and charge
transfer impedance is decreased. Coating
weight of around 1 wt% appears to provide the
best results for alumina coated NMC
electrodes.
In a similar manner to the graphite coating
above the cathode coating material should at
least match the purity of the cathode
compound which is normally synthesised from
4N+ precursors.
Similar to the graphite situation, cathode
purity is closely controlled and tightly specified
to be produced from 4N precursor materials.
AEM is currently developing a cathode coating
process where the alumina is formed on the
surface of the cathode particles from a 4N+
precursor